Too often I ask the class an organic chemistry question and I get this gem of an answer: “Ummm, is it because of resonance?” And when I ask my students to elaborate, they fail to do so. Cue the loud incorrect buzzer and awkward silence.
Students love to toss “resonance” around like it’s a magic word that fixes everything, but few actually get what it means. After dodging countless “resonance” grenades, I’ve decided it’s time to break this concept down properly. Otherwise, I’ll be buried under a landslide of these half-baked one-word answers.
When I think of “resonance”, I think of a cathedral and the sound of a bell resonating through its halls. Just as the bell's tone permeates every corner of the cathedral, resonance is characterised by its ability to spread and influence a larger area (delocalised), rather than remaining confined to a single location (localised).
This brings me to the term “delocalisation”.
What is delocalisation?
Delocalisation of electrons is when electrons move about several atoms, not staying in one fixed position. These electrons are not confined to a single ‘location’, hence the word ‘delocalised’.
Why does delocalisation happen?
The main driving force behind delocalisation is this – Electrons hate being confined. These little guys are happiest when they have room to roam and stretch their legs. This is because electrons are negatively-charged. When they are confined and squished together, they start to repel each other, making things tense and high-strung, resulting in higher energy levels. But give them some space, and there is less repulsion between them, resulting in lower energy levels.
Imagine a factory room fully packed with chickens – stuffy, sweaty and anxiety-inducing. High energy. Contrast this with a large spacious field – The chickens, with a lot of room to move about, feel comfortable, relaxed and at ease. Low energy.
Since delocalisation allows electrons freedom to move about a larger region, they stay at a lower energy level and the molecule in question will now be more stable. Therefore, delocalisation of electrons results in greater stability in a molecule.
Before we move on to the details…it is important that you first know this:
Only pi electrons delocalise
Not all electrons can delocalise. Only pi electrons can (recall that pi electrons are the electrons in a pi bond, which are formed through the side-on overlap of p orbitals). For example, in benzene, the pi electrons in the three pi bonds move while the sigma bonds stay intact. The curly arrows show the movement of the pi electrons, resulting in the resonance structure on the right (note that the double-headed arrow below ↔ is used to show that the two species are resonance structures of each other).
Electrons in a sigma bond do not delocalise. If electrons in a sigma bond could delocalise, that would mean breaking a single bond, which would mean changing the entire identity of the molecule.
Here is an incorrect example:
The sigma bond was wrongly moved, forming an entirely new molecule.
Delocalisation is NOT a chemical reaction, and hence should not change the identity of the molecule. Only pi electrons delocalise – and as to why, you will understand better as you read on.
So when does delocalisation happen?
Criteria for delocalisation
Delocalisation happens when the below two criteria are fulfilled.
1. The p orbitals in a molecule must be adjacent to each other.
p orbitals are like ‘hollow containers’ where pi electrons are housed. Pi electrons can jump from p orbital to p orbital, allowing them to freely move about. However, the pi electrons can only do so if the p orbitals are right beside (adjacent to) each other. Consider the following molecule A:
All the carbons are sp² hybridised and hence each has an unhybridised p orbital (refer to guide on hybridisation). Drawing in the p orbitals, we will have this diagram:
Since the p orbitals are right beside/adjacent to each other, they can overlap, allowing the pi electrons to jump from p orbital to the next p orbital, and to the next, and so on and so forth (represented by the arrow). I like to imagine the pi electrons as travellers hopping from inn to inn, finding a home in all of them.
Consider another molecule B:
One of the carbons is sp³ hybridised, and hence does not have an unhybridised p orbital. Drawing in the p orbitals, we will have this diagram:
Now, p orbital 2 and p orbital 4 are no longer adjacent to each other. The carbon in between them that does not have a p orbital, hence preventing pi electrons from jumping from p orbital 2 to p orbital 4. Hence, delocalisation cannot happen in this molecule, and the pi electrons are trapped in their respective double bonds.
Let’s refer to the diagram of p orbitals for the molecule A again. There is something I want to clarify.
The diagram here might mislead you to think that electrons jump from p orbital to p orbital, leaving one p orbital filled and one p orbital empty. However, a more accurate way of thinking would be that these electrons are constantly flowing through all the p orbitals, forming the following blue stream of electrons:
The blue stream of electrons shows that the electrons are flowing through all six adjacent p orbitals, forming a cloud of pi electrons, what we call a ‘pi electron cloud’.
You will find that you often use this term ‘pi electron cloud’ in your explanations involving resonance. A ‘pi electron cloud’ is essentially the delocalised pi electrons from all the double bonds involved, gathered in one cloud. Each double bond involved contributes 2 pi electrons into the cloud, and we end up with a ‘pi electron cloud’. For example, molecule A has 3 double bonds and hence has a pi electron cloud containing 6 pi electrons.
2. The p orbitals must be aligned.
For electrons to travel between p orbitals, the p orbitals must be aligned (parallel to each other). When the p orbitals are aligned, they can overlap effectively with each other, allowing pi electrons to jump from p orbital to p orbital. However, if the p orbitals are 90° to each other, they will not overlap, and electrons cannot jump between these orbitals.
Electrons jump from p orbital to p orbital the same way Mario jumps from pipe to pipe.
Mario can jump from pipe to pipe if the pipes are parallel to each other (aligned), but if they were 90° to each other, I can imagine Mario having a hard time.
Only p orbitals are involved in delocalisation
(TLDR: only p orbitals are involved in delocalisation. sp, sp² and sp³ hybrid orbitals are not involved in delocalisation.)
So far, we have only discussed delocalisation of electrons from p orbital to p orbital. Can delocalisation happen between p orbitals and sp³ hybrid orbitals? From the example of molecule B, we would be able to deduce that the answer is no.
molecule B:
We can visualise this with the below diagram of carbons 3 and 4 of molecule B. C3 is sp³ hybridised, so its sp³ orbitals are arranged accordingly in a tetrahedral shape. C4 is sp² hybridised, so its sp² orbitals are arranged accordingly in a trigonal planar shape, with one unhybridised p orbital (blue).
Notice that the blue p orbital is not aligned with any of the sp³ orbitals of C3. For them to be aligned, they have to be perfectly parallel to each other, lined up like soldiers. Therefore, the p orbital and sp³ orbitals are unable to overlap effectively with each other, and electrons cannot travel between them.
Can delocalisation then happen between p orbitals and sp² hybrid orbitals? For the same reason that they are not aligned, p orbitals and sp² orbitals cannot overlap and hence electrons cannot travel between them.
In the above diagram, the sp² orbitals (black) are in a trigonal planar shape and are all in the same plane. The p orbitals (blue) are perpendicular (90°) to these sp² orbitals and hence do not overlap with them. Instead, there will be orbital overlap between the two p orbitals since they are aligned.
Therefore, only p orbitals are involved in delocalisation. sp, sp² and sp³ hybrid orbitals are not involved in delocalisation.
(An exception to this is hyperconjugation, which is not in the H2 Chemistry syllabus.)
Since only p orbitals are involved in delocalisation, and p orbitals contain pi electrons, only pi electrons can delocalise. Conversely, since the sp, sp² and sp³ hybrid orbitals are not involved in delocalisation, and they contain sigma electrons, sigma electrons cannot delocalise.
Common examples of delocalisation
Benzene:
As every carbon in benzene is sp² hybridised, every carbon has a p orbital. Since the six p orbitals are adjacent to each other and aligned, they overlap side-on, allowing for the delocalisation of pi electrons. This forms a pi electron cloud, represented by the green and blue rings in the following diagram, kind of like donuts stacked on top of each other:
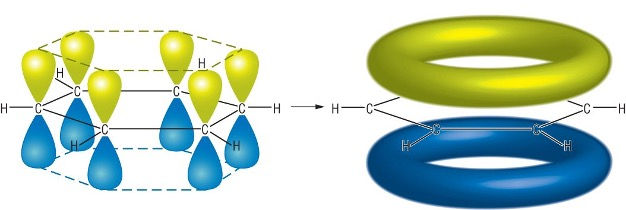
Since benzene has three pi bonds, each containing 2 pi electrons, the pi electron cloud formed consists a total of six delocalised pi electrons.
In 2D, we often see the delocalisation of pi electrons in benzene represented in the two resonance structures:
However, these two resonance structures are not exactly an accurate representation of how pi electrons move in benzene. At any one time, the molecule does not exist as 1a or 1b, but exists as a hybrid of both of them. This is because pi electrons are constantly moving throughout the molecule and are not stationary in their double bonds. This constant movement of electrons about the molecule is better represented by 1c, which shows the pi electrons circling about benzene in a ring.
Stability in benzene
The delocalisation of electrons in benzene makes benzene very stable. Remember? The pi electrons have more room to stretch their legs now. Instead of being confined to their respective double bonds, they can travel across all 6 carbon atoms. Like a huge playpen, how fun! Having more space will result in less repulsion between electrons, and therefore greater stability.
Benzene’s stability makes it very unreactive. It takes a lot of energy to break the bonds in benzene to form something else. Referring to the following diagram again, the delocalisation of electrons in benzene creates a structure with three rings stacked upon each other. Pick up three rubber bands and try pulling them apart. It requires way more force to break three rubber bands than just one.
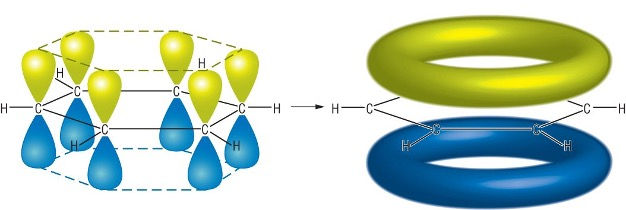
We will encounter more examples of delocalisation in Resonance Part II – stay tuned!
An exception: an example of unaligned p orbitals (allenes)
In the examples we have discussed so far, the p orbitals are always aligned. Let us now discuss an exception to this.
Consider molecule C:
In molecule C, the central carbon is sp hybridised, and hence has two unhybridised p orbitals. The two terminal carbons are sp² hybridised, and hence have one unhybridised p orbital each. Drawing in the p orbitals, we get such a diagram:
The two p orbitals on the central carbon are 90° to each other. Why is this so? Let’s recall that the p orbitals px, py and pz are always perpendicular to each other (see diagram below).
They lie on the x, y and z axes (which are perpendicular to each other), hence the names px, py and pz orbitals respectively. So if p orbital 3 was a px orbital, then p orbital 2 has to be either a py or pz orbital, which has to be 90° to p orbital 3.
Therefore, p orbitals 1 and 3 (blue) are aligned; and p orbitals 2 and 4 (orange) are aligned. There is overlap between p orbitals 1 and 3 and overlap between p orbitals 2 and 4.
However, the pair of p orbitals 1 and 3 are not aligned with the pair of p orbitals 2 and 4, and both pairs are perpendicular to each other. Therefore, pi electrons do not delocalise across all four p orbitals and are trapped in their respective double bonds.
Molecules like C are called allenes, compounds that have adjacent carbon-carbon double bonds. As the two pairs of p orbitals are perpendicular to each other, the two –CH2 groups are perpendicular to each other too. Therefore, allenes are not planar (see diagram below: white – H atoms, black – C atoms).
Comments